Due to the recent global pandemic there were a lot of recommendations for people to buy and test their oxygen levels with a device called a pulse oximeter. Naturally this leads to the question “What is pulse oximetry?”, and for the more curious you may also ask “How does it measure my blood oxygen with those little lights?”.
Pulse oximetry measures and record your pulse rate and the level of oxygen in your blood. The technology takes advantage of the fact that oxygenated blood is a different colour than non-oxygenated blood. Oxygenated arterial blood appears bright red, whereas the less oxygenated venous blood appears dark red/brown or purple.
Table of Contents
Pulse Oximetry is a clinical technique that gives doctors, nurses and other healthcare professionals a real-time, non-invasive access to heart rate and blood oxygen levels, two of the most important physiological health parameters for quick and timely general evaluations.
The ease of use of the application of the technology on a subject, a single clip on the finger, is quicker than manually assessing heart rate, and has the added benefit of being able to see how well oxygenated a person is allows health care workers to quickly establish whether the patient needs supplementary oxygen.
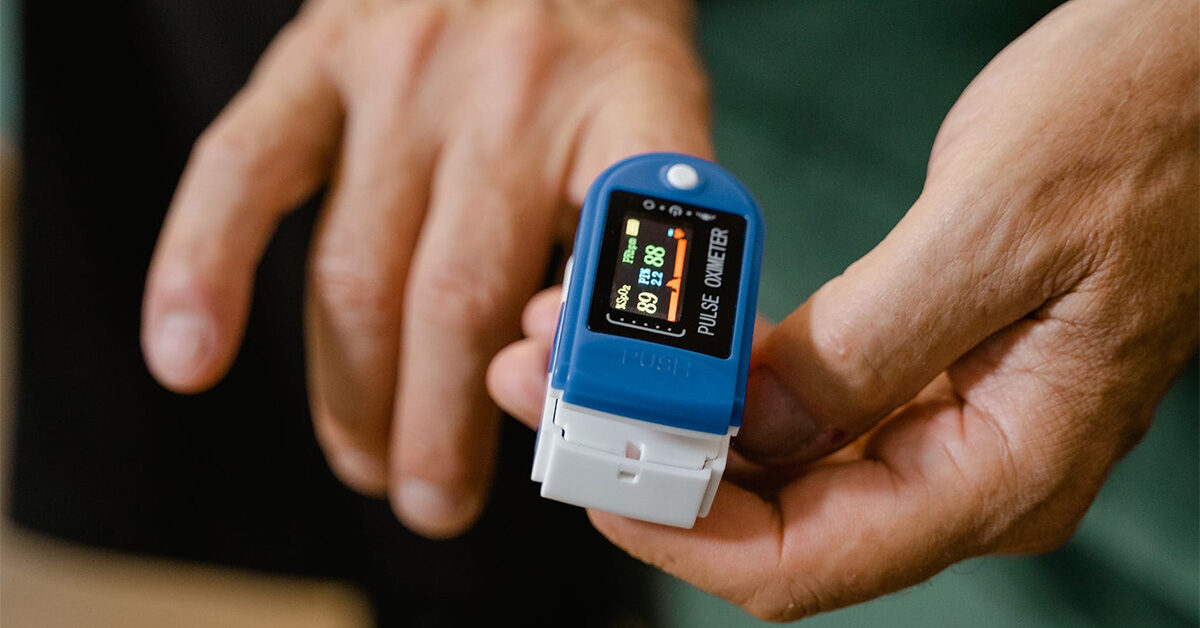
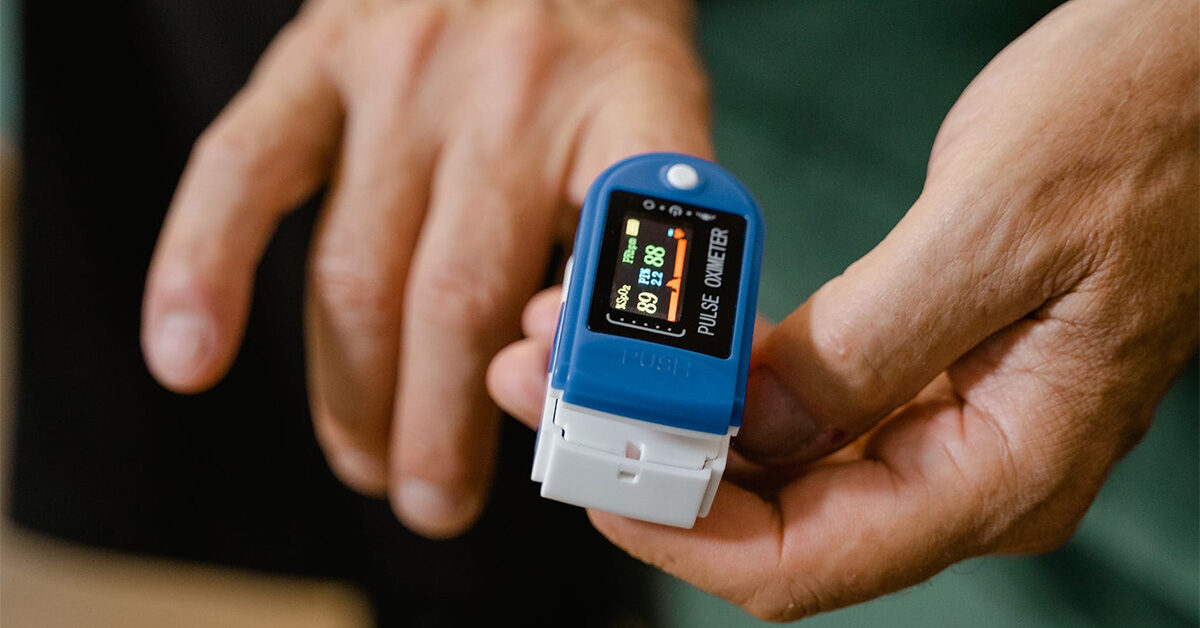
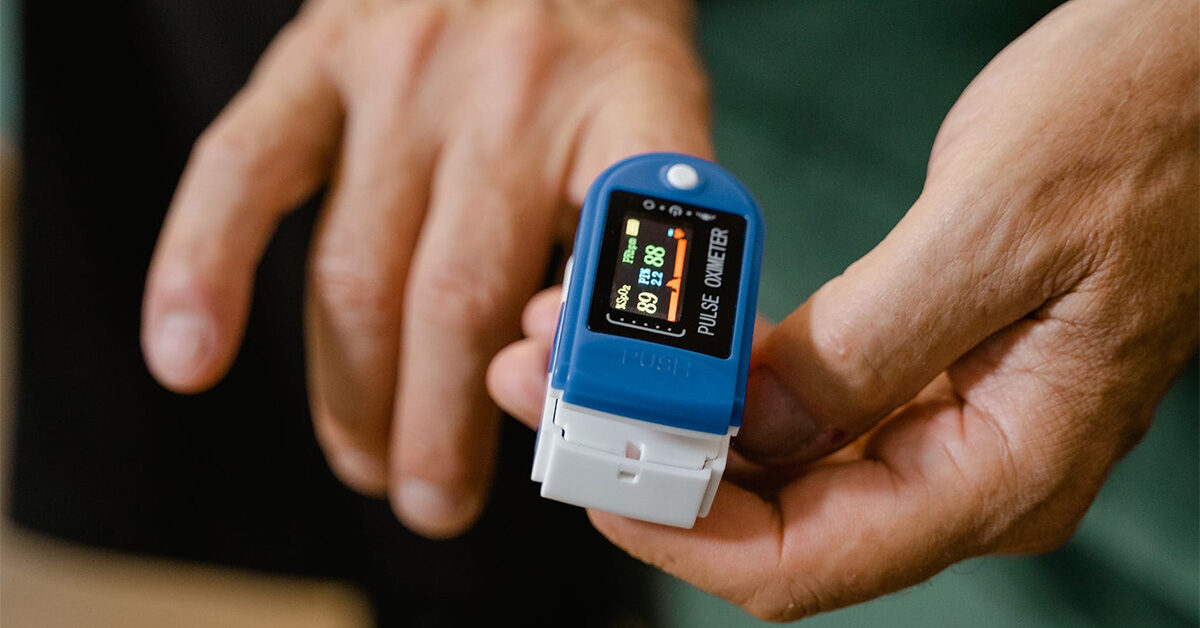
Assessment by a pulse oximeter is especially critical to emergency responders where victims of accidents may be compromised of oxygen, such as in house fires or when internal injuries may cause problems with the uptake of oxygen from the lungs to the blood.
How Does Pulse Oximetry Work?
Using a measurement technique called photoplethysmography the arterial blood can be sensed by specialised light sensors called photo diodes after the tissue being examined is illuminated with a light source, commonly a series of light emitting diodes (LEDs).
Importantly pulse oximetry is a dynamic light measuring technique that shines light of specific colours (wavelengths) into tissue and records how much of those colours are absorbed. Ideally the arterial blood should be as oxygenated as possible, i.e. close to 100% saturated.
The colour of the arterial blood is directly proportional to the amount of oxygen present, and because only the arterial blood has a strong “pulse” it can be separately measured from the remaining blood and tissue present in, say your finger.
Using Colour to Measure Oxygen
Blood is a substance of multiple light absorbers, and although it appears opaque, in fact transmits light over a broad range of wavelengths (colours) of light, and is especially reflective to red and infrared light. When talking about pulse oximetry the principle absorbers or contributors to the colour we see and are interested in are oxyhaemoglobin (HbO2) and haemoglobin (Hb).
When first observed by researchers it was noted that a significant colour change happened for Hb and HbO2 in the visible green part of the spectrum (535 – 560 nm) (Edsall 1972). This visualisation can be clearly demonstrated in the below image of the absorption colour spectrum for Hb and HbO2.
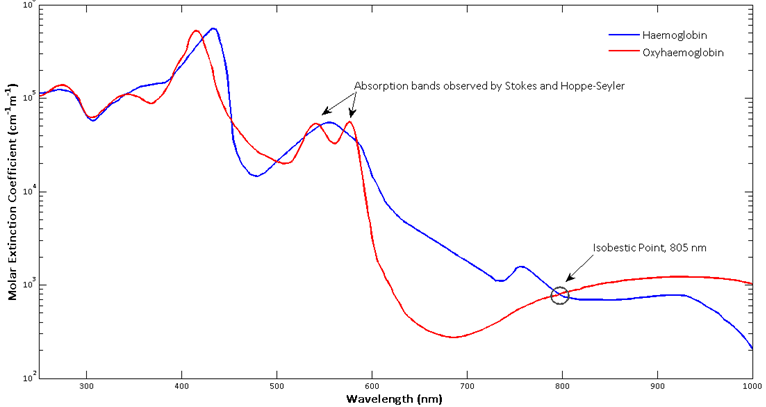
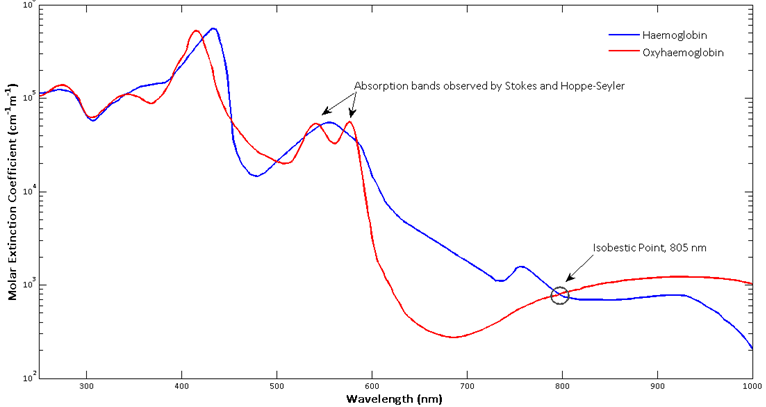
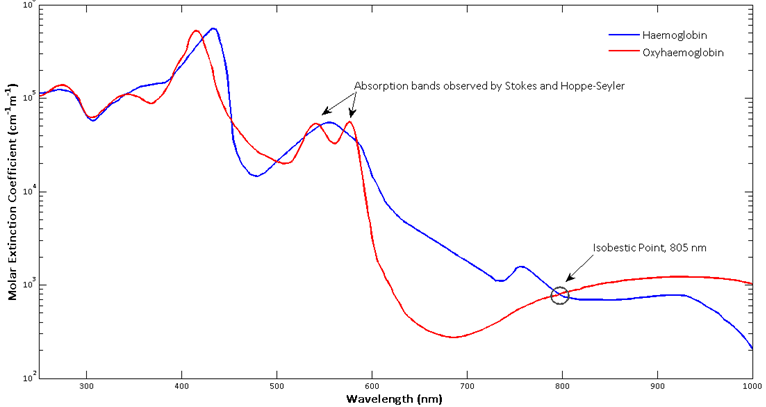
Although there have been oximeters that have used green light, modern pulse oximeters take advantage of the the red and near infrared region (630 – 950 nm). In his original experiments and developmental work of the pulse oximeter, the father of pulse oximetry, Takuo Aoyagi had selected a red (630 nm) and an infrared (900 nm) filter for his light sources. Light absorption at
630 nm was found to be most sensitive for oxygen saturation (Severinghaus, 2007), and 900 nm was chosen, because at the time Aoyagi was using an injection dye to measure cardiac output, which did not absorb this wavelength.
Using the Pulse to Select Arterial Oxygen Saturation
Takuo Aoyagi’s breakthrough moment for pulse oximetry was when he was observing his cardiac output experiments and noticed the pulsation of the PPG trace from his detector. Aoyagi realised that by computing the ratio of the pulsating (AC), and non-pulsating (DC), portions at two wavelengths (Red and Infrared) he could separate electronically just the arterial blood component from the rest of the blood and the surrounding tissue (Webster, 1997), thus solving the issue of early oximeters unable to, or employing complicated mechanisms to, remove the part of the PPG signal that was once the major source of error in previous experiments.
Before this breakthrough measurement sites were restricted to the earlobe, where previous incarnations of oximeters would temporarily and regularly occlude blood flow as a way of distinguishing between arterial blood and the rest of the surrounding tissue. Now they could be made nearly anywhere.
References
- EDSALL, J. T. 1972. Blood and hemoglobin: the evolution of knowledge of functional adaptation in a biochemical system, part I: The adaptation of chemical structure to function in hemoglobin. Journal of the History of Biology, 5, 205-257.
- SEVERINGHAUS, J. W. 2007. Takuo Aoyagi: Discovery of Pulse Oximetry. Anesthesia & Analgesia, 105, S1 -S4.
- WEBSTER, J. G. 1997. Design of pulse oximeters, Institute of Physics Pub.
Pingback: The History of Pulse Oximetry Measurement - Curious Cyborg
Pingback: What are those Lights on the Bottom of My Watch? - Curious Cyborg
Pingback: A Brief History and Origin of the Photoplethysmogram - Curious Cyborg